GreatMoves
General Relativistic Moving-mesh Simulations of Neutron-Star Mergers
The ERC Relativistic Astrophysics Group at the GSI Helmholtz Centre in Darmstadt focuses on hydrodynamical computer simulations of neutron-star mergers (see movie). The main goal of the group is to link obseravbles of neutron-star mergers to fundamental physics questions. This concerns for instance the properties of high-density nuclear matter and the transition to a quark-gluon plasma at finite chemical potential, which may be extracted from gravitational-wave signals of neutron-star mergers. The team also works on the formation of heavy elements through the rapid neutron-capture process in the ejecta of neutron-star mergers. These nucleosynthetic processes power the emission of kilonovae - the electromagnetic counterparts of colliding neutron stars. The team develops novel numerical methods to extract information about the underlying mass ejection and element formation from observations. The work of the group is supported by an ERC Starting Grant (grant agreement No. 759253). The team is lead by Priv.-Doz. Dr. Andreas Bauswein.
In October 2022 we received an ERC synergy grant together with colleagues in Copenhagen (D. Watson), Belfast (S. Sim) and Dublin (P. Dunne), which allows us to continue our research within a broader scope.
We offer Bachelor, Master and PhD projects (contact a.bauswein@gsi.de).
Research highlight: Moving-mesh simulations of neutron star mergers
Computational power is an issue when it comes to simulating complex phenomena like neutron star mergers in three dimensions. Hence, it is important to strive for novel numerical methods that allow to capture the violent dynamics of a neutron star collision and resolve the different spatial scales, on which different processes take place. A promising way forward are moving-mesh tools, where the computational grid moves with the fluid flow and thus the numerical resolution is adaptively placed where it is needed. Apart from these obvious advantages, such schemes also promise to be less prone to numerical artifacts because the advection across cell bounadries is minimized. Within a long-term development we have adapted and extended the moving-mesh code AREPO for simulations of relativistic systems. Our paper in MNRAS presents the first simulations of neutron star mergers with a moving-mesh code. Developing such a tool was one of the major goals of the GreatMoves project.


Research highlight: The Hyperon Puzzle in neutron stars
Hyperons are baryons that contain at least one strange quark. But they are not only strange, they are puzzling as well. The "hyperon puzzle " raised the question whether these articles should occur in the center of neutron stars. If so one could generally expect a softening of the neutron star equation of state. This however seems somewhat in tension with the observation of neutron stars with masses above two solar masses, which requires a certain stiffness of the high-density equation of state. In collaboration with colleagues from Barcelona we recently presented a comprehensive study of hyperons in neutron star mergers. We did not solve the hyperon puzzle, but we outlined possibilities to potentially identify the presence of hyperons in neutron stars by observing gravitational waves of the postmerger stage. If hyperons occur, the thermal pressure for a given amount of thermal energy is reduced. As consequence the merger remnant is more compact and gravitational wave frequencies slightly increased, which may then point to the presence of hyperons.
Research highlight: Forward modeling of kilonova spectra
A kilonova is the electromagnetic emission produced in the rapidly expanding explosion following a neutron star merger. What a telescope observes as kilonova many million light years away is the result of numerous atomic emission and absorption processes. Understanding the kilonova by modeling the emission requires to simulate all these atomic processes taking into account the properties of all different chemical elements in the expanding cloud. Shingles et al., ApJL 954, L41 presents such a simulation of radiation in three spatial dimensions. It includes the vast number of more than 40 million atomic transitions. By this detailed approach it is possible to trace back which chemical elements shape which part of the observed spectrum. For instance, the chemical elements strontium, yttrium and cerium seem to be quite important. It is remarkable that model reproduces prominent features of the kilonova AT2017gfo without fine-tuning the model to this particular observation. The calculation also highlights the important of three-dimensional kilonova models and makes predictions how kilonovae should look like from different observer angles.

Research highlight: Element production in neutron star mergers
Neutron star mergers produce heavy elements like gold and uranium through the rapid neutron-capture process. In fact, they are to date the only confirmed source where this process takes place. The nucleosynthesis occurs in the material ejected from the merger site; obviously only gravitationally unbound matter can be mixed into the interstellar medium and form new stars and planets. The ejection process during a neutron star coalescence is rather complex occuring over different time scales with different ejection mechanisms being involved. Simulations of neutron star mergers typically do not cover all mass ejection episodes, which obviously yields an only incomplete picture of the nucleosynthetic yields. In ApJL 951, L12 (2023) we have consistently included all mass ejection processes and computed the resulting element production and kilonova properties. For the specific models considered here, we observe that in comparison to a solar elemental distribution too little heavier elements are produced. This may suggest that this particular binary configuration may not be the most representative one and may only contribute a subdominant fraction to the total abundance (see left panel). The properties of the resulting kilonova are in remarkable agreement with the observed event in 2017 (see right panel).
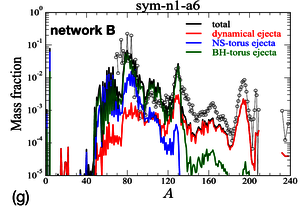

Research Highlight: Spherical kilonova explosion
The kilonova AT2017gfo associated with GW170817, the first neutron star merger detected by gravitational waves, has been a treasure trove for Nuclear Astrophysics. A kilonova emerges from the electromagnetic emission of the hot explosion of the material that is escaping the merger site. In these outflows heavy elements are formed and the nucleosynthesis process creating these elements heats the matter producing the electromagnetic emission. In a recent study, which was lead by colleagues from the Niels Bohr Institute, we investigated the geometry of the explosion. It came as a big surprise that the outflow is very spherical. This is a priori not obvious considering the large amount of angular momentum in the system, which stems from the orbital motion of the neutron stars. Hence, naively one would expect that the outflow features a pronounced symmetry axis instead of having the geomerty of a sphere. This observation is not only helpful to better understand kilonovae, but it may also be used as a new probe for cosmology to determine the Hubble constant. The analysis recently appeared in Nature. (See also the press release and our popular article in Sterne und Weltraum.)

Research Highlight: Dark Matter in Neutron Stars

Specific models of dark matter predict that it may accummulate in the gravitational potential neutron stars and form dark compact objects by self-interactions among the dark mMatter particles. The dark matter may be dragged along with the stars during a neutron star collision. We have simulated such a combined system of ordinary matter and dark matter (Physical Review D 107, 083002 (2023)). During a neutron star merger potential dark matter components remain bound to the system and reside in the central remnant without interacting with the baryonic matter other than gravitationally. As a result the dark matter components orbit in the central gravitational potential of the neutron star remnant and generate a long-lasting gravitational wave signal. Being fundamentally different from the signal produced by the baryonic matter, its specific signature can reveal the presence of dark matter and thus help to comprehend the properties of the still mysterious dark matter component of the Universe.
Research Highlight: Black-hole torus evolution

Material ejected into the interstellar medium by a neutron-star merger can be extremely massive and neutron rich and could therefore represent a major site of the rapid neutron-capture (r-) process that is responsible for the creation of many heavy elements, such as Gold and Uranium. Whether or not neutron-star mergers are a significant, or even dominant, source of r-process elements depends sensitively on the mass and level of neutron richness of the material released during and after the merger. A new study now investigated the conditions to achieve a high neutron density in outflows from a black-hole disk system, which is often left behind after a neutron-star merger. The study systematically clarifies the roles of individual weak interactions between neutrons, protons, electrons, and neutrinos for creating neutron-rich conditions. It is found that disks reach the highest neutron densities if they are massive enough for electrons to be degenerate, but not so massive for neutrino absorption to become dominant. These conditions happen to be realized exactly for disk masses in the range produced after typical neutron-star mergers. This provides strong support to the idea that neutron-star mergers are major r-process sites. (See also the press release).
Research Highlight: Black-hole formation in Neutron Star Mergers
Neutron stars are the densest stellar objects in the Universe. They cannot be arbitrarily massive because beyond some threshold the gravitational attraction becomes too strong and the gravitational collapse to a black hole is unavoidable. Therefore, the collision of two stable neutron stars can lead to the formation of a black hole. The outcome basically depends on the total mass of the system and whether or not it exceeds the threshold for black-hole formation. In a recent study, which appeared in Physical Review Letters, we investigated the outcome of neutron star mergers and under which conditions they result in a black hole. Interestingly, the exact threshold for the gravitational collapse depends sensitively on the properties of nuclear matter. In turn, these dependencies imply that the outcome of neutron star mergers can inform about still unknown properties of high-density matter. (See also the press release)

Research Highlight: Identifying a First-Order Phase Transition in Neutron-Star Mergers through Gravitational Waves
The transition from ordinary nucleonic matter to deconfined quark matter at higher densities is still mysterious. Possibly neutron stars feature a core of quark matter in their center. This offers the opportunity to learn about the hadron-quark pahse transition from observations of neutron stars. Based on computer simulations we identified an unambiguous signature of the presence of quark matter in the gravitational-wave signal of neutron-star mergers. The appearance of quark matter can be detected by comparing the signal of the premerger stage with the characteristic frequencies inferred from the postmerger phase. A significant increase of the postmerger gravitational-wave frequencies would be indicative of the occurrence of deconfied quark matter. The results of the study have been published in Physical Review Letters (see also the press release).
