Main research topics
Impact of low dose radon exposure on the organism

In LD-RT (low dose radiotherapy), photons or densely ionizing radiation, e.g. in radon spas, is used to treat rheumatoid arthritis, ankolysing spondylitis, musculoskeletal disorders, psoriasis and others (Figure 1). Cellular and molecular mechanisms underlying the observed alleviation from pain in radon spa are widely unknown.
GREWIS / GREWIS-alpha Consortium
Therefore, we set out to investigate potentially beneficial and harmful aspects of low dose radon exposure. To achieve this, we work together in the frame of the „GREWIS“-project with experts covering the fields of particle physics, cytogenetics and DNA repair, immunology, molecular cell biology and physiology, s. Helmholtz Association. The GREWIS-alpha consortium includes 7 working groups from 4 institutions and associated medical collaborators (Figure 2).
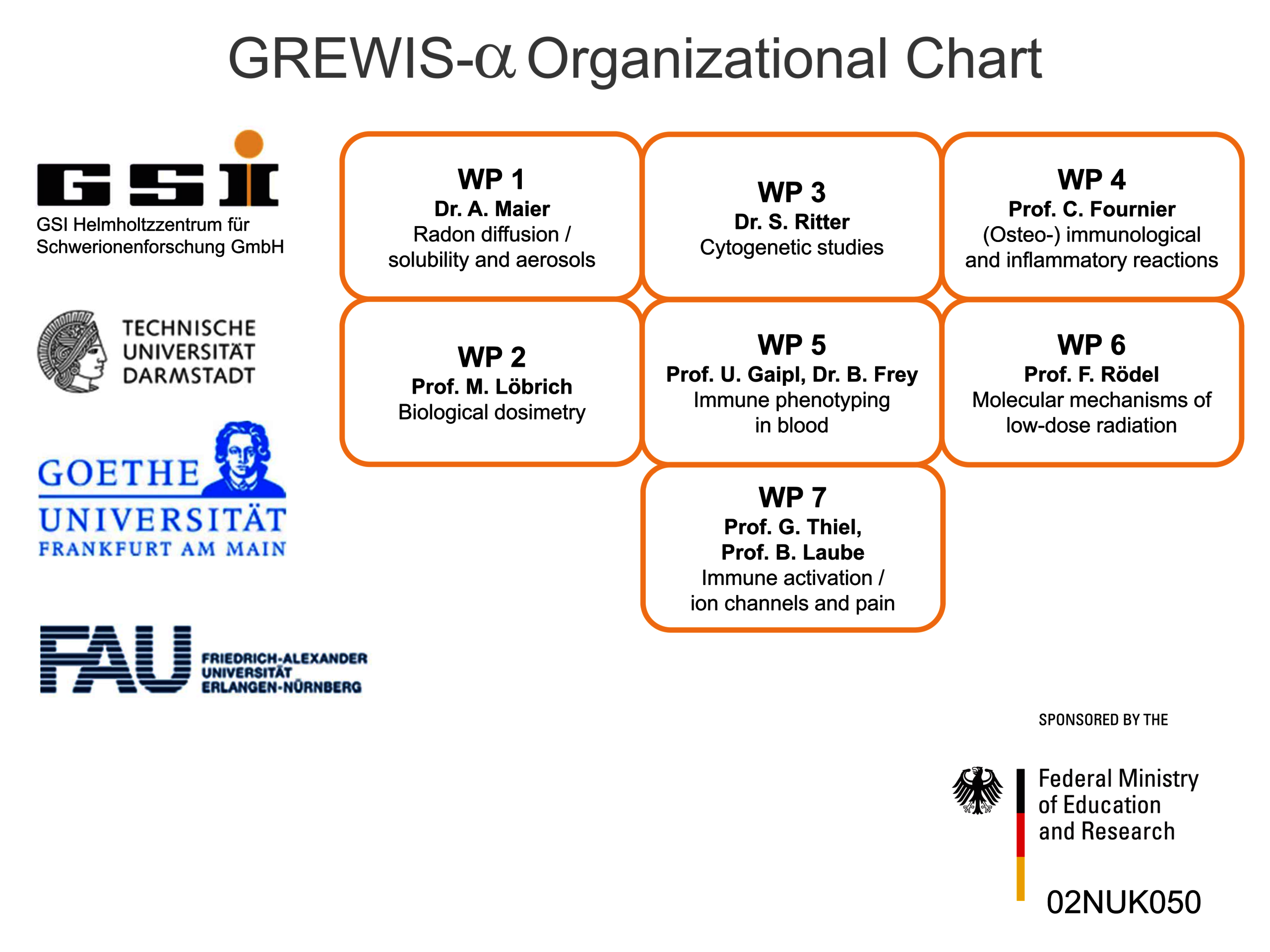
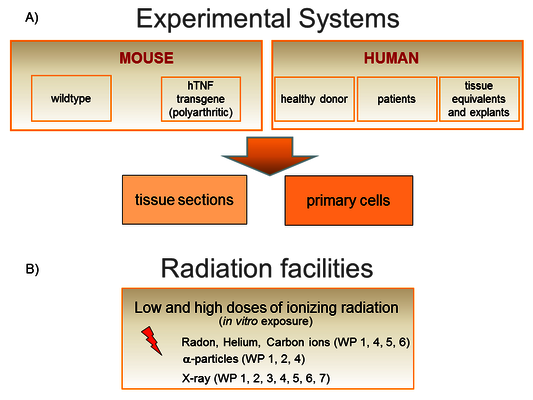

Prof. Dr. Claudia Fournier, Dr. Andreas Maier, Dr. Denise Eckert, Dr. Ayele Taddese Tsedeke
The research groups of the GREWIS projects have established an integrated strategy to study several aspects of risk and the potential immune-modulating effect of low radiation doses.
We work on a molecular and cellular level as well as in tissues and animals (Figure 3A). We have access to human bone marrow (hematopoietic and mesenchymal stem cells), skin from healthy donors, human knee tissue samples (infrapatellar fat pads and synovial membrane) and blood from patients undergoing radon therapy (RAD ‐ ON02, EudraCT 2016‐002085‐31) and low dose X-ray therapy (IMMO-LDRT; NCT02653079).
Furthermore, we have developed a unique tool for radon exposure: A chamber allowing radon exposure of cells and mice, operating under controlled conditions including humidity, temperature and radon concentration, which can be adjusted comparable to radon therapy or higher (Figure 4, click to enlarge) [1]. Diffusion and solubility measurements of radon and an analysis algorithm were developed [2]. Furthermore, using molecular dynamics simulations, an uneven distribution of radon between fatty acids and isotonic saline solution could be explained mechanistically [3].
In addition, an α-particle irradiation setup has been constructed and is available at GSI and TU Darmstadt. To this end, an Americium-241 source with comparable energies to the α-particles emitted by radon is used. With this source, cells can be irradiated on thin foils for defined time intervals [4].
Furthermore, heavy ions with similar characteristics as α-particles (helium and carbon ions) are used (Figure 3B). In order to be able to estimate the dose and thus also the risk of radon exposure, measurements were carried out on a volunteer patient after therapy in a radon gallery. The evaluation models developed here can be applied to other applications, for example for dose estimation in a mouse model or in physical samples.
Biodosimetry based on DNA damage markers after radon exposure has been established for different organs, and X-ray reference curves have been measured.
As blood vessels as well as the interaction of endothelial cells of the blood vessel wall and hematopoietic cells have a crucial role in the first steps of immune reactions, a “flow chamber” was constructed to mimic the shear stress caused by the blood flow.
Experiments are performed in vitro using both primary mouse and human cells (hematopoietic, skin, bone, synovial and fat cells; differentiated from stem and progenitor cells from healthy donors), also as 3D tissue equivalents.
An in vivo approach takes advantage of the hTNFα-transgenic mouse model and the K/BxN serum transfer model for polyarthritis to investigate maturation and activity of immune and bone cells.
Our first RAD-ON01 study revealed that patients have reduced pain after radon treatment. This could be due to a decrease in the number and activity of bone-resorbing osteoclasts and has also been confirmed in the patient study on photon radiation (IMMO-LDRT). Additionally, in the plasma of radon and LDRT patients, a decrease in collagen fragments and a lower activity of an enzyme involved in bone resorption (TRAP) could be detected. In a second ongoing RAD-ON02 study, a cross-over design was used for the first time, i.e. there is a control group and a group of radon-exposed patients for two consecutive years with the same 100 patients. With this design, a possible placebo effect should be eliminated. After a year, the patients are treated again, but the groups are swapped. The study is blinded, i.e. it is not known neither to patients, doctors nor to researchers which group received which treatment.
This is consistent with functional changes observed for low dose photon exposure (LDRT) in the polyarthritis mouse model and results obtained in vitro on the molecular level (Figure 5, click to enlarge) [5-14].
The current state of knowledge on the possible effect and risk of radon therapy was summarized and published in a review [15].

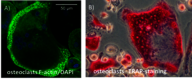
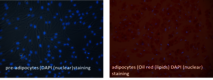
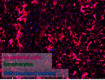
Immunogenicity and immunomodulation of radiation
Dr. Alexander Helm
In contrast to the putative anti-inflammatory and immune suppressive effects of low doses on the organism, higher doses of radiation can trigger immune reactions that can improve the treatment outcome. Such reactions can be useful particularly in the therapy of metastatic cancer disease and hence combination of radiation and immunotherapies represents a powerful tool. In our research we investigate the response of (radiation-resistant) tumor cells to radiation with low and high LET (linear energy transfer, photons versus particles), with the aim of supporting the killing of tumor cells by activating an immune response [16, 17]. In an in vivo model system (osteosarcoma, metastases in the lungs), we were able to demonstrate that carbon ions – in combination with immunotherapy (so-called checkpoint inhibitors), and also alone – could fight lung metastases more effectively than photons used in comparison [18]. Our current research activities are devoted to checking these promising results in other (human) cell lines as well as clarifying the mechanisms involved and how carbon ion therapy can be beneficial. We investigate this using various in vitro and in vivo models.
Carbon ion radiotherapy (CIRT) alone leads to a reduction of lung metastases in an osteosarcoma in vivo model, which is not the case for conventional photon therapy (XRT), when compared to untreated negative controls (NC). Addition of immunotherapy (Figure 8: a mix checkpoint inhibitors, CPI) fosters the immunogenic response, leading to further reduction of lung metastases. This shows the vast potential for CIRT as a match in combination with immunotherapy in terms of checkpoint blockade [17].
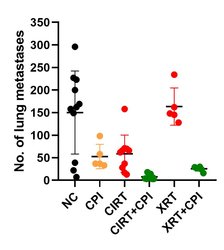
Radiation response of human hematopoietic stem and progenitor cells
Dr. Daniela Kraft
Secondary cancer occurs months to years after exposure and is an important issue in radiation protection, for radiotherapy and manned space missions. The interference in the process of self-renewal and differentiation of hematopoietic stem and progenitor cells (HSPC) via radiation exposure is considered to increase the risk for radiation induced leukaemia (rAML). However, risk assessment for radiation induced leukemogenesis needs an improved knowledge about the radiation response of HSPC and, in particular, their genetic stability. Therefore, we investigated the transmission of chromosomal changes in HSPC exposed to low and higher LET irradiation (up to 85 keV/µm). In view of known differences in genetic stability and DNA repair between murine and human HSPC, we used human HSPC, provided in collaboration with Prof. H. Bönig (Frankfurt University) (Figure 10, click to enlarge). Our results showed, independent of LET, no chromosomal instability, but a considerable frequency of clonal chromosomal aberrations in the descendants of irradiated HSPC [19, 20]. To elucidate the capacity for correct DNA repair in HSPC, we assessed in collaboration with Prof. L. Wiesmüller (Ulm University), the quality and molecular components of DSB (double strand break-) repair compared to mature peripheral blood lymphocytes using an EGF plasmid reporter systems (developed by Prof. L. Wiesmüller). We could show that HSPC bear a deficiency for DSB repair (homologous recombination) due to diminished NF-κB signaling, which was confirmed for heavy ion exposure and could explain the occurrence of clonal aberrations in the following generations after exposure [21, 22]. More recently, we started to investigate the combined influence of micro-gravity and high LET exposure in HSPC.
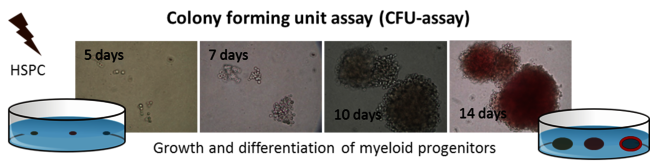
Space radiation induced neuroinflammation
Dr. Felicitas Rapp
For longer manned space missions, for example a mission to Mars, it is important to know about risks for the astronauts. In our project, we investigate the radiation induced neuroinflammation, and if it can be reduced by test substances. We are funded by ESA and DLR. To induce neuroinflammation, we use an organotypic hippocampal slice culture model from mice. These slice cultures are irradiated with heavy ions occurring in space (iron ions, Fe) ex vivo and treated with our test substances afterwards (Fig. 11 A+B). At several timepoints up to 29 days after irradiation, samples are taken and analyzed regarding an inflammatory reaction. Inflammation in the brain is mediated by the innate immune cells, microglia, so they are in focus in this project. We developed a system where microglia are stained and their inflammatory activation and morphology are analyzed by confocal microscopy (Fig 11 C+D). This project is a collaboration with Dr. Sonja Kallendrusch and Prof. Ingo Bechmann (Institute of Anatomy, University of Leipzig). In the next step, we will use a mouse model based on our findings, and test the effect of space irradiation in combination with a treatment with our test substances on their cognitive performance. In addition, we will test new shielding materials in collaboration with Prof. Chiara LaTessa (University of Trento, Italy)

Heavy ion induced late effects in tissue
Dr. Julia Wiedermann
In other projects, our research group studies late effects induced by exposure to heavy ions. Radiation induced non-cancer effects include impact on the functionality of tissues and organs, which is often caused by loss of functional cells or modifications in the differentiation process and subsequent reorganisation of the tissue. The resulting changes can evoke inflammation, a physiological “repair” process, which may turn into a chronic process, as in the case of fibrosis. The increasing application of charged particles in radiotherapy requires a better understanding of these processes for charged particles, in particular for carbon ions.
Especially skin is exposed in all scenarios of radiation exposure. We investigate in another project the early skin response after carbon ion exposure, whose molecular and cellular basis is not well understood. In order to complement previous RBE data sets obtained in a pig model [23] by cellular and molecular studies, we use mono- and co-cultures of epidermal cells, a full skin 3D tissue equivalent, and ex vivo exposed human skin from healthy donors, provided by our collaboration partners PD Dr. M. Podda and M. Kovacs (Darmstadt Hospital) (Figure 12, click to enlarge). Similar to photon exposure, epidermal tissue organization was modified at low doses and differentiation at high doses of carbon ions, with a moderate release of inflammatory cytokines [24]. In ongoing experiments, the results are verified in carbon ion irradiated pig skin.

Theses pig skin samples were obtained in experiments performed in collaboration with MD I. Lehmann and MD D. Packer (Mayo Clinics, Rochester), dedicated to investigate the possibility to treat cardiac arrhythmias by targeted charged particle irradiation. In a first step, isolated pig hearts were irradiated, and cellular and molecular changes were analysed [25]. Then, pigs were irradiated with carbon ions to demonstrate the efficiency of targeted small volume irradiation of structures in the heart which are responsible for electrophysiological conductivity. We then analysed the induced damage in the targeted and non-targeted areas (with Dr. P. Simoniello, Parthenope University of Naples) [29]. (Figure 13, click to enlarge)
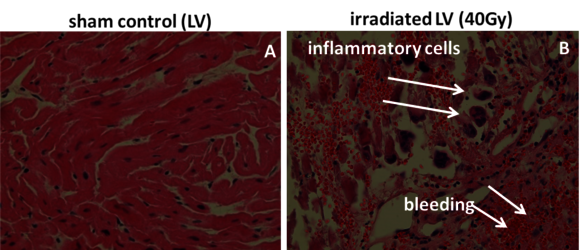
References:
[1] Maier et al., Nucl. Instr. Meth. Phys. Res. B, 362:187-193 (2015)
[2] Maier et al., Nucl. Instr. Meth. Phys. Res. B, 416:119-127 (2018)
[3] Sanjon et al., Scientific Reports 9,10768 (2019)
[4] Maier et al., Int. J. Radiat. Biol, 96:2, 206-213 (2020)
[5] Large et al., Rad. Oncology, 9:80 (2014)
[6] Large et al., Strahlenther Onkol, 191:742-749 (2015)
[7] Thangaraj et al., Chemico-Biological Interactions, 259B: 49-412 (2016)
[8] Roth et al., Pflügers Archive-European. J. Physiol. 467:1835-1849 (2014)
[9] Gibhardt et al., Scientific reports, 5:13861 (2015)
[10] Rühle et al., Autoimmunity, 50(2):133-140 (2017)
[11] Erbeldinger et al., Front Immunol., 8:627 (2017)
[12] Cucu et al., Front Immunol., 8:882 (2017)
[13] Maier et al., Nucl. Instr. Meth. Phys. Res. B, 416:119-127 (2018)
[14] Rühle et al., Modern Rheumatology, 29(1):165-172, (2019)
[15] Maier et al., Int. J. Mol. Sci. 22(1), 316 (2021)
[16] Ebner et al. 2017, Front Immunol., 8:99 (2017)
[17] Helm et al. 2018, Int J Part Ther., 5:84-93 (2018)
[18] Helm et al. 2021, Int J Radiat Oncol Biol Phys., 109:594-602 (2021)
[19] Kraft et al., Mutat. Res., 777:43-51 (2015)
[20] Becker et al., IJRB, 85(11):1051-1059 (2009)
[21] Kraft et al., Leukemia, 29:1543-1554 (2015)
[22] Rall et al., Frontiers in Oncology, 5:250 (2015)
[23] Zacharias et al., Acta Oncologica, 36(6):637–642 (1997)
[24] Simoniello et al., Frontiers in Oncology, 5:294 (2016)
[25] Lehmann et al., Circ Arrhythm Electrophysiol. 8(2):429-38 (2015)
[26] Rödel et al., Front Immunol. 8:519 (2017)
[27] Durante et al., Trends Mol. Med., 19(9):565–82 (2013)
[28] Shreder et al., Int. J. Mol. Sci.19(9): 2717 (2018)
[29] Rapp et al., Sci Rep 9(1):5000 (2019)
To read more:
- Rühle et al., Autoimmunity. 50(2):133-140 (2017)
- Rödel et al., Front Immunol. 8:519 (2017)
- Erbeldinger et al., Front Immunol. 8:627 (2017)
- Cucu et al., Front Immunol. 8:882 (2017)
- Eckert et al., Cells 11:72 (2022)
- Deloch et al., Cells 11:689 (2022)
- Papenfuss et al., Radiat Environ Biophys 61:279-292 (2022)
- Eckert et al., Front Immunol. 13:817281 (2022)
Press releases
- Frankfurter Allgemeine Zeitung 27.07.2021: Doppelte Attacke auf den Tumor
- Darmstädter Echo 15.07.2021: Kombi-effekt gegen Krebszellen
- Pressemitteilung GSI/FAIR 24.06.2021: TRON nutzt GSI-/FAIR-Experimentierzeit für Krebsforschung: Kombination von Schwerionentherapie und mRNA-Impfstoff
- Physics World, Particle therapy Research update (Tami Freeman) 24.11.2020: Carbon ions team up with immunotherapy to tackle advanced tumours
- Pressemitteilung GSI/FAIR 09.11.2020: Perspektiven der Tumorbehandlung: Forschende untersuchen Kombination von Kohlenstoffionen- und Immuntherapie
- European Space Agency 18.02.2019: Pillen für das Space_brain
- ARD Planet Wissen 15.02.2019: Radon – Gefährliche Strahlung im Alltag. Radon-Therapie
- Darmstädter Echo 28.11.2017: Experimente im Heilstollen
- Pressemitteilung GSI 19.01.2017: Forschungen zur Radontherapie gehen weiter. Neues Projekt wird mit vier Millionen Euro gefördert
- Spiegel 17/2016 vom 23.04.2016: Schön verstrahlt
- Leschs Kosmos | ZDF - Sendung vom 05.04.2016: Documentation über Tschernobyl
- Pressemitteilung GSI 05.08.2015: Erforschung der Radon-Therapie geht weiter
- Auswärtiges Amt 04.04.2014: Schmerzfrei dank Radioaktivität (Video)
- Frankfurter Allgemeine Sonntagszeitung 17.02.2013: Es gibt sie doch, die gute Radioaktivität
- Pressemitteilung GSI 29.02.2012: Wie und warum wirkt eine Radontherapie?