The discovery of element 110 at GSI – a report by Sigurd Hofmann, an element discoverer
Extract from the speech by Sigurd Hofmann, element discoverer from GSI, given at the baptism of the element darmstadtium (from the naming ceremony brochure of the element darmstadtium that appeared on December 2, 2003).
‘The search for element 110 was surely the most thrilling and exciting experiment in the research careers of everyone involved.’

In this talk I would like to give you an impression of that time.
Step 1: Selecting the best reaction. During the early years of GSI and starting with the known elements 104 through 106, we gradually worked upward to the new elements 107, 108 and 109 using targets of lead and bismuth. The projectiles available were isotopes of titanium through to iron.
As a consequence, the projectile for the synthesis of element 110 was the nickel isotope with mass number 62. The advantage of this choice was that we changed as little as possible in the reaction itself. This way we could hope to keep the error margin low when extrapolating into the unknown. Additionally, the resultant evaporation-residual – that is, the nucleus that we were ultimately proving and that is formed from the vaporization of a neutron from the hot compound nucleus – would change through its radioactive decay by radiating an alpha particle into nuclei and elements that are already known. Thus, an absolutely reliable procedure for identifying the unknown initial decay.
Step 2: How great is the probability of formation, or, to put it another way, how much beam time do we need?
To answer this question we were able to refer to the cross-sections previously measured for elements 102 to 109. For element 110, we extrapolated with high probability a value of 1.5 pb. So far so good, but what did this mean? For one atom of element 109 we needed 14 days of beam time. The cross-section there was 10 pb. It followed that for 1.5 pb we would need about 100 days, and that was without allowance for statistical fluctuations, for which we would have to allow a factor of three. In other words, a year’s worth of beam time, day and night, without a weekend off, and no holidays. That might have been possible as an exception, but Herr Kienle (head of GSI from 1984 to 1992, editor’s note) would surely not have granted us such a long beam time, as GSI was also involved at that time with another experiment that caused sensation, the search for the positron.
‘We had to make the equipment more sensitive so that the measurement time could be reduced from 365 days to just 35 days.’
So what were we to do? It was quite simple. We had to make the equipment more sensitive, to cut the measurement time by an order of magnitude – down from 365 days to just 35 days. This happened from 1988 to 1993. The main steps, without going into detail, were as follows: On the accelerator side a new high-charge state injector was constructed that allowed the intensity to be increased by a factor of three. On the SHIP side we increased the reliability, transmission and detection sensitivity by building a new target wheel, making changes to the separator and developing a new detector system including detection electronics. An important component of this, the time-of-flight detector, was provided by our new partner from Bratislava.
The beam from the UNILAC collides with a lead nucleus in the target – a very rare event. Of these few events, in most cases the projectile and target nucleus separate again after exchanging a few nucleons, or else the resultant compound nucleus decays through splitting. Only a tiny fraction of them survive through the evaporation of a neutron and emission of gamma radiation. All this happens extremely quickly within the target itself, which is only 0.4 micrometers thick, and although the composite system is moving at 3% of the speed of light. A fantastically complex process of nucleus interaction. The SHIP separator separates the evaporation residual from the projectiles within a microsecond. It is implanted into the detector system at the output of the SHIP and is finally and uniquely detected by its decay.
There are various different versions of how a fusion reaction can proceed.

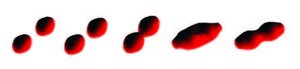


[...] After what has been shown it becomes clear why one parameter preoccupied us in particular: what was the correct projectile energy? To determine this we examined the excitation curves previously measured – that is, the yield as a function of beam energy. These measurements are tiresome, particularly where the cross-sections are small. For this reason the excitation curves were measured only incompletely. To determine the correct energy for element 110 using the data shown is little more than a guessing game. The situation is further complicated by the fact that most of the reaction theories then current required an extra push of energy if heavy nuclei were to fuse at all. The choice of the energy for elements 108 and 109 was influenced by this prediction. As was soon to become evident, this was an error with serious consequences. To extrapolate the correct energy for element 110 we proposed to our user committee, which is responsible for assigning beam time, that we would measure the excitation curves for elements 104 and 108 before carrying out the main experiment. We expected element 104 to be completed relatively quickly, as its cross-section, at 10,000 pb, is very high. For element 108 there was only one measurement point. But, we said to ourselves, if we want to find element 110, it must also be possible to measure an excitation curve for element 108. Otherwise we would do better to give up our work there and then.
‘Unfortunately, another entirely insurmountable obstacle then appeared.’

What we needed as the projectile for element 108 was enriched iron-58. Our accelerator people estimated a quantity of about four grams for a four-week run; one gram then cost some US$500,000. This would have bankrupted the GSI budget completely, and again we were facing the end of our superheavy research. Luckily, however, a very warm relationship had meanwhile developed with our Russian colleagues in Dubna, which was further aided by the fall of the Iron Curtain. We were therefore not shy to ask casually whether our Russian colleagues, with their famously inexhaustible sources of raw materials, might be able to help us. They could.
‘Over US$10 million in one small pile.’
For an earlier experiment our colleagues Sasha Eremin and Andrey Popeko had brought with them a precious sample of iron: 20.7 g of enriched iron-58. We spread the treasure out on my desk, took a photo and could hardly believe our eyes. Over US$10 million in one small pile. The experiment had been saved. To honor the donor, Yuri Oganessian, that evening we upended a few bottles of French red wine. Not Russian, you may say, but one must never overdo things. Now things began to move very fast. In June the excitation curve for rutherfordium was measured. A clear maximum was obtained for the 1n channel at 15.3 MeV excitation energy. We saw the 2n channel and eventually also, much reduced through increasing fission, the 3n channel. Then in October, for the measurement with hassium, the Russian iron was used. We began with the measurement point at 20 MeV, which was the old value with which element 108 was discovered in 1984. A week later we had only measured one more atom. So how could we hope to obtain an excitation curve at that rate? We decided to change the energy, and, going against theoretical prediction, we set the UNILAC to a lower energy level. That same evening our detectors were showing the first two results; after four days we had 12 ‘events’, and the cross section was 10 times higher than previously.
‘The first bets were placed’
Now the way forward was clear, and no extra push of energy was required. The first bets were placed on how high the maximum would be. We decided to collect the difference amount in German marks [the currency in use at the time]. The total raised was 300 marks, and we celebrated our success at a Thai restaurant. By the end we had produced 75 atoms of the hassium-265 isotope. We had gained valuable information about the alpha decay of hassium, the daughter to be expected from the decay of element 110. And the most important outcome: the maximum of the cross-section was at 13.8 MeV, thus 1.5 MeV lower than for element 104. From this data we extrapolated an optimal excitation energy for the production of element 110. This was about 13.0 MeV. On Monday, November 7, 1994 the accelerator was changed from iron to nickel. Everything ran perfectly from the outset. For Thursday, Gottfried had invited our employees out for a trip on the Rhine. When I arrived at the institute at about noon that day I was greeted by Fritz Hessberger and Victor Ninov with a clap on the shoulders and the words: ‘We’ve got it.’ That morning they had evaluated the magnetic tape from the previous day, and indeed, on Wednesday, November 9 at 4:39 pm, the first nucleus of element 110 was measured.
‘By about three o’clock in the morning the first draft of an announcement was ready.’
The three of us examined the measurement data closely and came to the conclusion that this was the proof of the first atomic nucleus of element 110 with mass number 269. And that had happened after only two days of measurement time. What were we to do now? Should we shout it from the rooftops? We decided to refrain. For one thing, our colleagues were out that day, and for another, we would then have become embroiled in all kinds of discussions for a long time. Quite apart from this, we urgently needed time to write an official announcement. We knew after all from our Russian colleagues that there was another group in Dubna that had been looking for element 110 since September, but had been irradiating plutonium rather than lead. We had always had to be prepared to deal with ‘news’ from Dubna. It was a matter of urgency. To keep it brief, by about three o’clock in the morning the first draft of an announcement was ready. We placed copies on our colleagues’ desks and finally got some sleep.
The final version was submitted to the Zeitschrift für Physik journal on November 14 in Heidelberg. In the photo we see the team of discoverers with the first decay chain of element 110. And almost all of us who were involved have come back together today. We are particularly pleased that the only woman in the picture, Frau Ursula Vogel, who was our secretary, is also here with us. By the end of the beam time two other decay chains had been measured, before the projectile beam was changed on November 21, 1994 from nickel-62 to nickel-64. With these, a further isotope of element 110, with mass number 271, was synthesized and proven. Now the probability of formation had increased by a factor of four. It is this isotope with which our work on element 110 was just recently confirmed at RIKEN in Japan and at the Lawrence Berkeley Laboratory. Finally, on December 1 the target was changed from lead to bismuth, ready for the search for element 111. This search was made possible by deferring a positron experiment to the new year, enabling us to prolong our beam time by three more weeks. This was a fortunate change to the beam time schedule, as it was rewarded with three decay chains of the new element 111. But that is another story.
‘Surely the most exciting of our lives.’
When we finished on December 22, 2½ months of beam time lay behind us – surely the most exciting of our lives. What remained for us to do was to think of names for the new element. We wanted to be prepared for when the IUPAC would invite us to propose a name. The internal meeting of the SHIP group took place on December 10, 1997. Believe me, we found it difficult to have to leave out many good suggestions. Still, by the end of the meeting we were in agreement, and as usual with special events, a number of bottles of red wine marked the occasion. French again, you understand.
I think that with ‘darmstadtium’ we have given element 110 a name that would also have pleased the founders of SHIP and the UNILAC, Heinz Ewald and Christoph Schmelzer. To a large extent we owe them our gratitude for being able to celebrate today. And in this spirit we would wish to continue our research work on the superheavy elements in the future, hopefully with continued success, with yet greater detection sensitivity, on an international level as before and with a growing number of young physicists of both genders.’